Over the past three decades, astronomers have discovered planets orbiting Sun-like stars throughout the universe. This discovery ended 2,500 years of debate about whether worlds existed beyond our solar system, but it came with a shock. The most common kind of planet in the universe is the type of world that doesn’t exist in our small corner of the cosmos: what astronomers call “Super-Earths” and “Sub-Neptunes,” planets with much greater masses than ours and which could, in theory, sustain life.
Astronomers concluded a little over a decade ago that every star in the night sky hosts a family of worlds. Importantly for the search for life, one in five of those stars will have a planet in the “Goldilocks” zone (sometimes referred to as the “habitable zone”). That’s the band of orbits that are just right for water to pool up on a planet’s surface into puddles, lakes and oceans. It’s a good bet that life needs liquid water to form, so the discovery of Goldilocks worlds (of which there are billions) pushed the search for extraterrestrial life to the front of science’s to-do list.
Planet hunting across interstellar distances is spectacularly difficult. At first, all scientists could learn from their telescopes was the size and mass of newly discovered worlds. That was enough to see that the galaxy’s average planet — the kind that appears most often in most solar systems — looks nothing like the worlds orbiting our Sun.
Our solar system has two kinds of worlds. In the inner solar system, there are “terrestrial” worlds — Mercury, Venus, Earth and Mars. These are basically spherical cakes of layered metal and rock; their cores mostly iron and nickel; and their outer layers made of rock-stuff — silicon, oxygen and magnesium.
Further out in the solar system lie “giant” worlds. There are the gas giants Jupiter and Saturn, which weigh more than 100 times Earth’s mass and are mostly made of hydrogen and helium. Then come the “ice giants” Uranus and Neptune, which are surrounded by layers of frozen slush made of ammonia, methane and other compounds.
Terrestrial worlds and giants. That’s it for our solar system. There’s no planet orbiting our Sun with a mass between that of terrestrial Earth and ice giant Uranus (which is fourteen times the mass of Earth). In the wider universe, however, the most common type of planet lies in that empty space between these two forms. These worlds are known as Super-Earths and Sub-Neptunes.
With billions of these planets strewn across the galaxy, their ability to host life becomes an urgent question. Are Super-Earths just scaled-up versions of our world requiring scaled-up versions of Earth life? Or are they something else entirely?
Answering this has put scientists under pressure. That’s not a figure of speech — understanding Super-Earths and Sub-Neptunes means understanding pressure. A planet’s gravitational pull increases with depth. At the Earth’s core, the gravity squeeze reaches a million times that at the surface. Those high pressures deep in our planet’s interior aren’t inconsequential. They set the conditions for life at the surface, including the presence of tectonic plates, which shaped evolution, and magnetic fields, which shield us from dangerous solar radiation. What happens below shapes what’s possible above. All the extra mass inside Super-Earths and Sub-Neptunes means their interior squeeze is driven to extremes that scientists don’t understand.
How does rock behave deep in a Super-Earth when it’s crushed 100 times harder than inside Earth? Will it still flow slowly, like road tar on a hot day, creating something similar to the Earth’s drifting continents? Or are tectonic plates a scientific impossibility on Super-Earths? How does ice behave when it’s subject to the insane pressures deep in a Sub-Neptune?
Directly exploring the extreme pressures in Super-Earths and Sub-Neptunes pushes scientists to their own extremes. Traditional laboratory methods can’t reach pressures 100 times higher than Earth’s core, so we can’t replicate what’s happening on the surface of these distant worlds. Facing that limit, planetary scientists have joined forces with plasma physicists who run giant lasers at places such as the Laboratory for Laser Energetics in upstate New York (where I’m a scientist). These city block-sized lasers were initially developed to study nuclear fusion.
The work has already borne fruit. High-intensity laser light was recently used to compress iron to Super-Earth pressures, and gives us a clue about the temperature at which solid iron at the center of a Super-Earth will melt into liquid form. Swirling currents of liquid iron at the Earth’s center are what give our planet its magnetic field — a field that deflects harmful particles from the Sun. The test indicates that Super-Earths might host their own life-protecting magnetic fields.
The extreme interiors of Super-Earths represent only half the problem for understanding these mystery worlds. If we want to know about the surface conditions where life can form, astronomers also need to understand their atmospheres.
The Earth probably didn’t start with the thin but life-giving veil of gases we enjoy today. When it first formed, it had a molten rock surface (a “magma ocean”) which was probably surrounded by a thick atmosphere of hydrogen and helium gas. But those elements are so light that Earth’s gravity couldn’t hold them for long, and they quickly bounced off into space. Only later, as the magma ocean cooled, gases like carbon dioxide and nitrogen were pushed out of the newly solidified rock to form a “secondary atmosphere.” (The oxygen we breathe came billions of years later.)
Super-Earths and Sub-Neptunes probably started the same way. But because they’re so much larger, it’s possible they have held on to their original atmospheres. When it comes to life on these worlds, everything depends on whether they have or haven’t.
Unlike our planet, most Super-Earths should have enough gravity to hold on to their big puffy primordial hydrogen atmospheres. Here’s the important point: hydrogen is a powerful greenhouse gas, far better at trapping solar energy than, say, carbon dioxide. A hydrogen atmosphere is so good at holding in heat that the surface of these worlds can end up hotter than a pizza oven. Not a great place for life. But astronomers have observed that many planets with Super-Earth masses appeared not to have a big, puffy extended atmosphere.
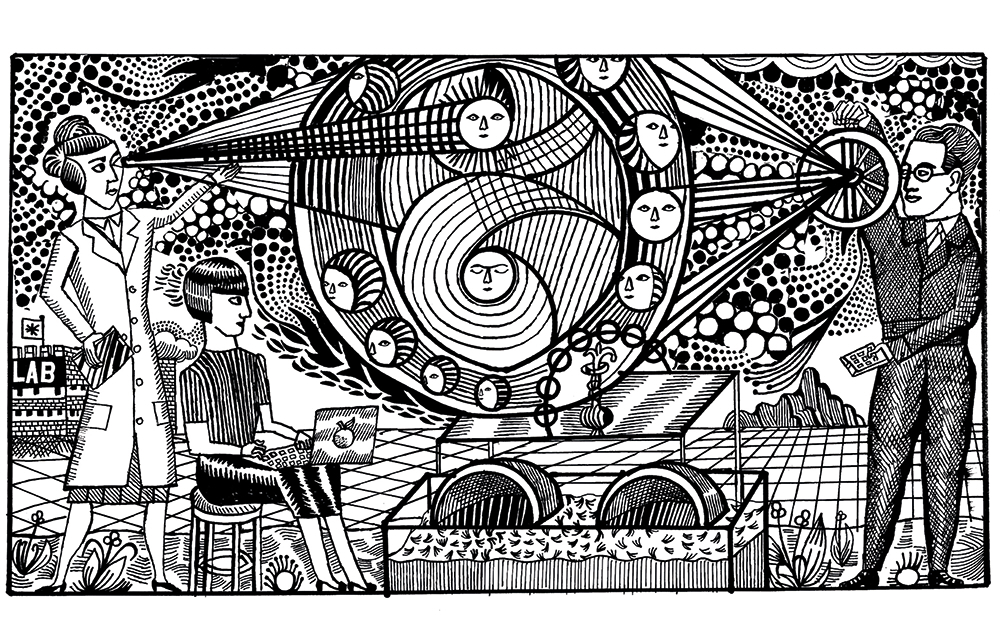
So where did the hydrogen go? The culprit appears to be the parent star, whose radiation can strip a Super-Earth of its hydrogen veil. This would suggest Super-Earths are habitable. If they can push out secondary atmospheres as the Earth did billions of years ago, then perhaps their surfaces might end up hospitable for life.
Sub-Neptunes provide more atmospheric mysteries. The Cambridge astronomer Nikku Madhusudhan and his colleagues recently made the radical proposal that some Sub-Neptunes might be “Hycean Worlds.” Madhusudhan gave this name to planets with hydrogen atmospheres and vast liquid water oceans on the surface (Hydrogen Ocean = Hycean).
Madhusudhan realized that the enhanced greenhouse warming from hydrogen atmospheres can change everything that we’d expect about “habitable zones.” By trapping so much solar energy in a hydrogen layer, planets far away from the host star can still exist in a warm liquid state. If so, then Hycean Worlds would constitute an entirely new class of habitable planet.
Using the James Webb Space Telescope, Madhusudhan’s team recently found evidence that K2-18b, a Sub-Neptune orbiting a red dwarf star in the constellation of Leo, showed strong evidence of being a Hycean World. It’s a huge planet with eight times more mass than Earth and its ocean would be equally impressive. Earth’s seas go no deeper than seven miles, but if K2-18b is Hycean then its oceans would cover the entire planet, reaching depths of 500 miles. If 500-mile-deep Hycean World oceans exist, then the question becomes: can life exist in dark seas where the pressure would be millions of times higher than on Earth’s ocean floor?
No one knows. Some questions about Super-Earths and Sub-Neptunes worlds may require the next generation of giant ground-based telescopes, which are being built now. These monsters will have mirrors almost as large as football fields. The effort will eventually pay off. Super-Earths and Sub-Neptunes are the most alien of alien worlds, because we didn’t even know they existed until recently. After contemplating our solar system for thousands of years, we’re suddenly confronted with how limited our vision has been. But these worlds will soon become familiar, and if we aren’t alone, at some point so too will their inhabitants.
This article was originally published in The Spectator’s September 2024 World edition.
Leave a Reply